Overview
Ultrasound has been primarily used as a medical imaging technique, allowing doctors to visualize internal body structures, as well as fluids and blood flow in the body to detect anomalies. While ultrasound is familiar as a diagnosis tool, targeted pulses of ultrasound is an emerging therapy that offers a minimally invasive alternative to traditional surgical approaches for the treatment of neurological disorders. In particular, recent studies have demonstrated that magnetic resonance-guided low-intensity focused ultrasound (FUS) serves as a potential treatment for Alzheimer’s disease (AD) by reversibly opening the blood-brain barrier (BBB) for localized delivery of therapeutics.
How does focused ultrasound work?
Ultrasound refers to sound waves of frequency higher than 20 kHz, which is inaudible to the human ear. As the waves pass through the tissue, the tissue experiences alternating periods of high pressure (compression) and low pressure (rarefaction). The mechanism of FUS is analogous to using a magnifying glass to focus beams of sunlight on a single point to burn a hole in a leaf. FUS uses an acoustic lens to converge sound waves precisely to a focal point with millimeter accuracy while keeping the surrounding tissues intact. While the tissues being penetrated by ultrasound are not affected, the convergence of multiple beams of FUS energy elicits important biological effects at the target zone, creating the possibility of treating various medical disorders without the need for incisions. This technique is often used in conjunction with magnetic resonance, which identifies and targets the tissue to be treated, as well as monitors and guides the procedure in real time (Izadifar et al., 2020).
The blood brain barrier - A major challenge for treating Alzheimer’s disease
Alzheimer’s disease is the most common form of dementia and its prevalence is projected to triple by 2050 (Hebert et al., 2013). AD is characterized by progressive memory and cognitive decline, and the major pathological hallmarks of AD are the accumulation of the toxic extracellular amyloid beta (Aβ) proteins and intracellular neurofibrillary tangles composed of hyperphosphorylated tau protein in brain tissues. Abnormal levels of Aβ clump together to form plaques between neurones, while the neurofibrillary tangles inside nerve cells disrupt the transportation mechanism of neurons, sabotaging the synaptic communication between neurons. AD is currently a non-curable disease, with the only approved drugs merely providing symptomatic relief, but does not stop the disease from progressing.
A major obstacle in the treatment of such neurological disorders is the presence of the blood-brain barrier, a layer of endothelial cells connected through tight junctions, adherens junctions and gap junctions, that envelops the blood vessels of the brain. Normally, the BBB plays a crucial role in protecting the central nervous system by impeding harmful substances, such as toxins and pathogens, from transiting from the blood to the brain parenchyma. However, when it comes to the treatment of diseases, this protective guard of our brain can become our worst enemy, as nearly 98% of therapeutic drugs and molecules are unable to bypass the barrier (Pardridge, 2020). This poses a significant challenge for the brain to achieve sufficient bioavailability of therapeutics for the treatment to be effective.
Overcoming the blood brain barrier
To overcome the BBB, pharmaceutical treatments require systemic dose escalation or invasive procedures like convection-enhanced delivery and direct intracranial infusion. However, these approaches are risky and might contribute to side effects such as infection or blood clots.
Recent advances have led to a surge of interest in FUS as a minimally invasive approach to achieve BBB opening with lower surgical risks and shorter recovery. Coupled with ultrasound contrast agents, also known as microbubbles, FUS has been demonstrated to disrupt the BBB transiently in a controlled, safe and targeted manner, improving drug delivery to the brain. The mechanical effects of FUS, namely cavitation, is the key to BBB disruption. Since large amounts of energy are required for FUS to induce cavitation on its own, the high energies may cause mechanical or thermal injury to the vessel or parenchyma.
To address this problem, microbubbles are incorporated into FUS treatment. Microbubbles are small (1–10 µm) lipid or protein shelled bubbles filled with an inert gas, and they are injected intravenously prior to the start of each sonication. Under the influence of ultrasonic fields, these circulating microbubbles enter a stable state of oscillation, expanding during rarefaction and contracting during compression (Timbie et al., 2015). This applies mechanical stress on blood vessel walls and causes transient separation of endothelial tight junctions that cement the BBB within seconds, and with a high degree of specificity and accuracy. Intravenous administration of microbubbles can significantly reduce the energy needed to disrupt the BBB since the ultrasound no longer needs to generate the bubbles on its own, reducing the integrity of the BBB without hemorrhage, unintended tissue damage or other adverse side effects. The increase in BBB permeability persists for approximately 10 to 24 hours, temporarily opening the gate for the delivery of medications to deep brain regions such as the hippocampus and the entorhinal cortex (Rezai et al., 2020).
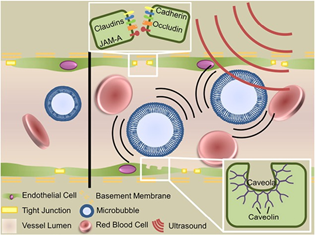
Delivering therapeutics to the brain
This innovative ultrasound technique has opened the door for efficacious therapeutic treatments like intravenous immunoglobulin (IVIg) immunotherapy. IVIg is a blood product derived from the pooled plasma of healthy human blood donors, it contains naturally occurring antibodies against Aβ peptides. FUS-induced BBB permeability enables IVIg to circumvent the BBB and reach the hippocampus, an area in the brain involved in learning and memory, and is severely degenerated in AD patients. Increasing the bioavailability of IVIg to the hippocampus has shown promise in reducing Aβ pathology. The treatment has resulted in a significant reduction in Aβ plaque load, specifically plaque number and size.
Yet, the mechanisms of actions of IVIg contributing to reduced plaque burden remains elusive. It is postulated that antibodies contained in IVIg exert their functions by binding to Aβ and inhibiting their aggregation. Another potential mechanism is supported by the “peripheral sink” hypothesis (the idea that levels of soluble Aβ in the brain and the periphery are in equilibrium). According to this hypothesis, IVIg binds to circulating Aβ in blood such that peripheral depletion of Aβ leads to efflux of Aβ from the brain (Menendez-Gonzalez et al., 2018).
In addition, it is also suggested that IVIg protects neurons against Aβ‐induced neuronal apoptosis by attenuating cell-death pathways. Mitogen-activated protein kinase (MAPK) signaling pathways such as p38, JNK, and NFκB signaling cascades are induced by cellular stress and are correlated with neuronal death. Activation of these pathways may trigger cell death by phosphorylating transcription factors regulating cell death. IVIg administration effectively prevents neuronal apoptosis by inhibiting cell death pathways and by elevating levels of the anti‐apoptotic protein Bcl-2 (Widiapradja et al., 2012).
Apart from reducing Aβ plaque pathology, IVIg-FUS is also demonstrated to dampen excessive inflammation and increase neurogenesis (the generation of new neurons from adult neural stem cells). IVIg-FUS reduces hippocampal levels of proinflammatory cytokine TNF-α, which is known to inhibit neurogenesis, promote Aβ production, and impair synaptic plasticity thus inducing memory impairment. This treatment is shown to potentiate adult hippocampal neurogenesis through cell proliferation, maturation, and survival, a process that plays a crucial role in memory formation and preserving cognitive functions.
FUS-BBB opening allows improved access for IVIg from the blood to the hippocampus, where it reaches therapeutic efficacy at a relatively low dosage. For the use of IVIg in clinical trials, lowering the effective dose required for efficacy can lessen the burden on the availability of IVIg as a human source biologic which is in high demand for treating several neurological diseases (Dubey et al., 2020).
Treatment with FUS alone reduces Alzheimer’s disease pathology
Interestingly, it was discovered recently that FUS alone, without the delivery of therapeutics, also significantly decreases Aβ plaque deposition. It is postulated that the glymphatic system might be kicked into gear via FUS, enhancing the clearance of Aβ. The glymphatic system is a fluid-movement and waste-clearance system that is unique to the brain. Its mechanism relies on the interchange of cerebrospinal fluid (CSF) and interstitial fluid (the fluid surrounding the brain’s cells) that allows waste products in ISF to be transferred to CSF and transported out of the brain (Lee et al., 2020).
Contrast-enhancement dyes were used and observed with MRI scans to monitor the changes in the brain following the FUS treatment. The images revealed the dye moving along the glymphatic efflux pathway. This suggested that FUS itself may modulate fluid movement patterns and elicit immunological responses along this system, enhancing the drainage of toxic Aβ from the brain parenchyma to CSF and then lymphatics (Aryal et al., 2020). It is also plausible that FUS induces the infiltration of phagocytic immune cells into the brain, which can aid in Aβ plaque clearance (Poon et al., 2018). Such tantalizing results unlock new insights into the physiological mechanism by which FUS modifies brain amyloid levels, as well as inspire new treatments to this debilitating disease.
Looking forward
In sum, microbubble-enhanced FUS treatment offers a scalpel-free alternative to traditional invasive approach to induce temporary BBB opening, addressing one of the major challenges in neurology. Alternative therapeutics aimed at the clearance of tau proteins and Aβ plaques are currently under study. Coupled with FUS and the subsequent BBB opening, administration of monoclonal antibodies, such as RN2N (Janowicz et al., 2019), aducanumab (Sevigny et al., 2016) and donanemab (Shi et al., 2021), shows promising results in addressing the pathological hallmarks of AD. Not only does FUS allow focal delivery of therapeutics to deep brain regions, it also holds the potential for stimulating clearance of abnormal protein aggregates by targeting glymphatic pathways. Hopefully in the near future, FUS technology can be utilized to slow down, or even reverse the progression of AD.
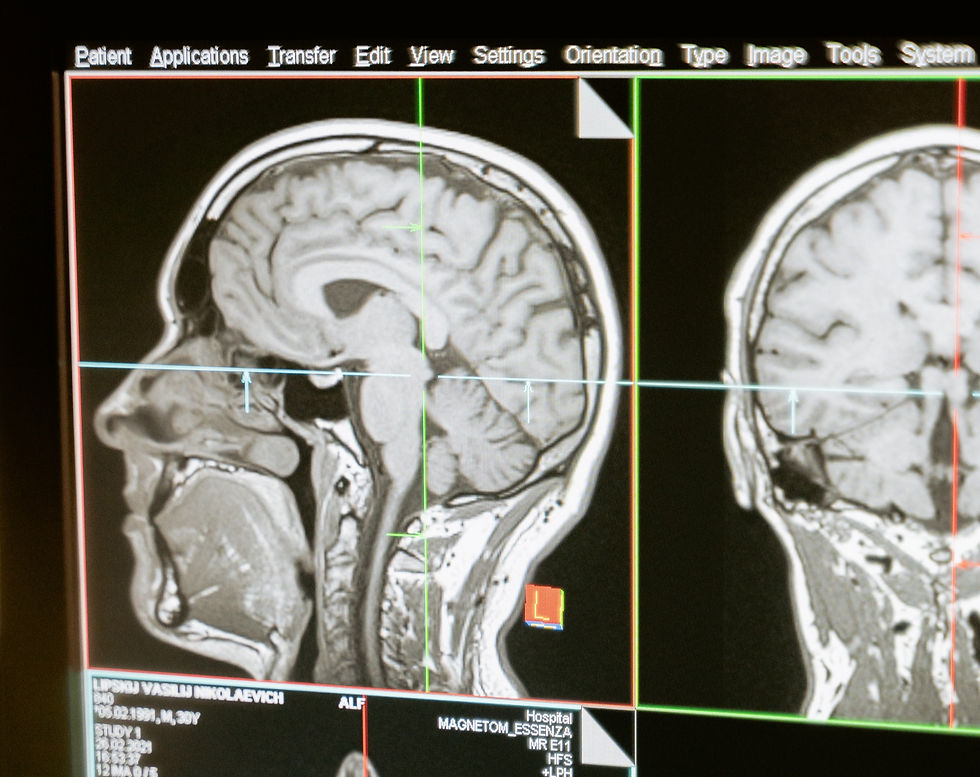
Bibliography
Izadifar, Z., Izadifar, Z., Chapman, D., & Babyn, P. (2020). An Introduction to High Intensity Focused Ultrasound: Systematic Review on Principles, Devices, and Clinical Applications. Journal of Clinical Medicine, 9(2), 460. https://doi.org/10.3390/jcm9020460
Hebert, L. E., Weuve, J., Scherr, P. A., & Evans, D. A. (2013). Alzheimer disease in the United States (2010–2050) estimated using the 2010 census. Neurology, 80(19), 1778–1783. https://doi.org/10.1212/wnl.0b013e31828726f5
Pardridge, W. M. (2020). Blood-Brain Barrier and Delivery of Protein and Gene Therapeutics to Brain. Frontiers in Aging Neuroscience, 11, 20. https://doi.org/10.3389/fnagi.2019.00373
Timbie, K. F., Mead, B. P., & Price, R. J. (2015). Drug and gene delivery across the blood–brain barrier with focused ultrasound. Journal of Controlled Release, 219, 61–75. https://doi.org/10.1016/j.jconrel.2015.08.059
Rezai, A. R., Ranjan, M., D’Haese, P.-F., Haut, M. W., Carpenter, J., Najib, U., Mehta, R. I., Chazen, J. L., Zibly, Z., Yates, J. R., Hodder, S. L., & Kaplitt, M. (2020). Noninvasive hippocampal blood−brain barrier opening in Alzheimer’s disease with focused ultrasound. Proceedings of the National Academy of Sciences, 117(17), 9180–9182. https://doi.org/10.1073/pnas.2002571117
Menendez-Gonzalez, M., Padilla-Zambrano, H. S., Alvarez, G., Capetillo-Zarate, E., Tomas-Zapico, C., & Costa, A. (2018). Targeting Beta-Amyloid at the CSF: A New Therapeutic Strategy in Alzheimer’s Disease. Frontiers in Aging Neuroscience, 10, 2–3. https://doi.org/10.3389/fnagi.2018.00100
Widiapradja, A., Vegh, V., Lok, K. Z., Manzanero, S., Thundyil, J., Gelderblom, M., Cheng, Y.-L., Pavlovski, D., Tang, S.-C., Jo, D.-G., Magnus, T., Chan, S. L., Sobey, C. G., Reutens, D., Basta, M., Mattson, M. P., & Arumugam, T. V. (2012b). Intravenous immunoglobulin protects neurons against amyloid beta-peptide toxicity and ischemic stroke by attenuating multiple cell death pathways. Journal of Neurochemistry, 122(2), 321–332. https://doi.org/10.1111/j.1471-4159.2012.07754.x
Dubey, S., Heinen, S., Krantic, S., McLaurin, J. A., Branch, D. R., Hynynen, K., & Aubert, I. (2020). Clinically approved IVIg delivered to the hippocampus with focused ultrasound promotes neurogenesis in a model of Alzheimer’s disease. Proceedings of the National Academy of Sciences, 117(51), 32691–32700. https://doi.org/10.1073/pnas.1908658117
Lee, Y., Choi, Y., Park, E.-J., Kwon, S., Kim, H., Lee, J. Y., & Lee, D. S. (2020). Improvement of glymphatic–lymphatic drainage of beta-amyloid by focused ultrasound in Alzheimer’s disease model. Scientific Reports, 10(1), 3. https://doi.org/10.1038/s41598-020-73151-8
Mehta, R. I., Carpenter, J. S., Mehta, R. I., Haut, M. W., Ranjan, M., Najib, U., Lockman, P., Wang, P., D’haese, P.-F., & Rezai, A. R. (2021b). Blood-Brain Barrier Opening with MRI-guided Focused Ultrasound Elicits Meningeal Venous Permeability in Humans with Early Alzheimer Disease. Radiology, 298(3), 654–662. https://doi.org/10.1148/radiol.2021200643
Poon, C. T., Shah, K., Lin, C., Tse, R., Kim, K. K., Mooney, S., Aubert, I., Stefanovic, B., & Hynynen, K. (2018). Time course of focused ultrasound effects on β-amyloid plaque pathology in the TgCRND8 mouse model of Alzheimer’s disease. Scientific Reports, 8(1), 1. https://doi.org/10.1038/s41598-018-32250-3
Janowicz, P. W., Leinenga, G., Götz, J., & Nisbet, R. M. (2019). Ultrasound-mediated blood-brain barrier opening enhances delivery of therapeutically relevant formats of a tau-specific antibody. Scientific Reports, 9(1), 1. https://doi.org/10.1038/s41598-019-45577-2
Sevigny, J., Chiao, P., Bussière, T., Weinreb, P. H., Williams, L., Maier, M., Dunstan, R., Salloway, S., Chen, T., Ling, Y., O’Gorman, J., Qian, F., Arastu, M., Li, M., Chollate, S., Brennan, M. S., Quintero-Monzon, O., Scannevin, R. H., Arnold, H. M., . . . Sandrock, A. (2016). The antibody aducanumab reduces Aβ plaques in Alzheimer’s disease. Nature, 537(7618), 50–56. https://doi.org/10.1038/nature19323
Shi, Q., Sun, T., Zhang, Y., Power, C., Hoesch, C., Antonelli, S., Schroeder, M. K., Caldarone, B. J., Taudte, N., Schenk, M., Hettmann, T., Schilling, S., McDannold, N. J., & Lemere, C. A. (2021). Ultrasound-mediated blood-brain barrier disruption improves anti-pyroglutamate3 Aβ antibody efficacy and enhances phagocyte infiltration into brain in aged Alzheimer’s disease-like mice. BioRxiv, 10–11. https://doi.org/10.1101/2021.01.15.426806
Comments